Understanding Atmospheric Carbon Storage Mechanisms
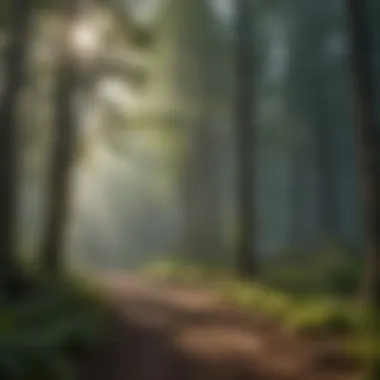
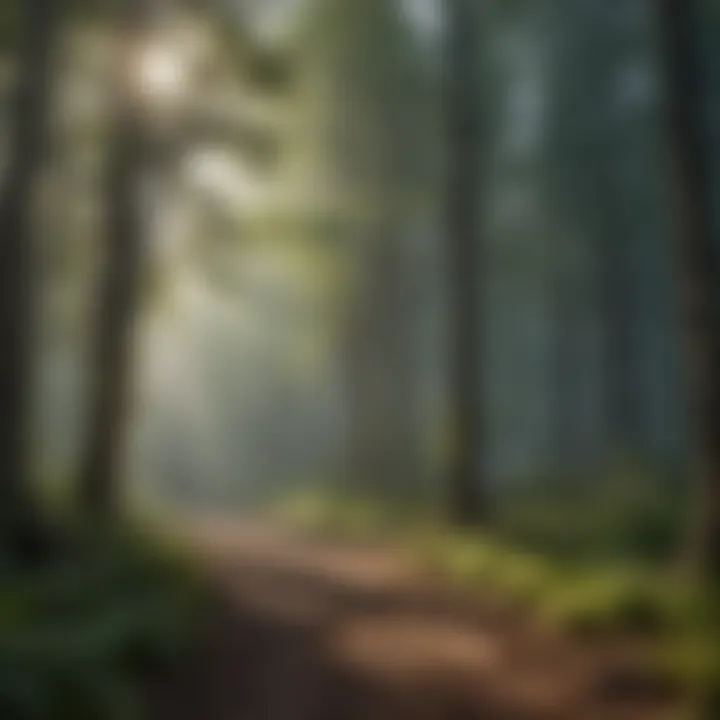
Intro
Understanding Forestry Practices
Forestry practices play a vital role in carbon storage within the atmosphere. Managing forests sustainably ensures that they continue to act as significant carbon sinks. This section will cover different aspects of forestry practices that can enhance or inhibit carbon storage.
Types of Forestry Practices
There are two main types of forestry practices: traditional forestry and sustainable forestry.
- Traditional Forestry: Often focused on immediate economic gain, these practices can lead to degradation of forest ecosystems. Deforestation and high-intensity logging reduce the capacity of forests to sequester carbon.
- Sustainable Forestry: In contrast, sustainable forestry takes a long-term view. This method emphasizes maintaining the health and biodiversity of forests while extracting resources. It encompasses practices such as selective logging and reforestation.
Historical Context
Historically, forests have been managed with various objectives in mind, including timber production and land clearing for agriculture. The impact of such practices has been profound, contributing to significant carbon emissions. Understanding the lessons learned from historical forestry can inform modern approaches to forest management, especially in the context of climate change.
Principles of Woodland Stewardship
Woodland stewardship principles guide sustainable practices that enhance carbon storage, ensuring that forests provide ecological benefits.
Conservation Techniques
Adopting effective conservation techniques is crucial for maintaining healthy forest ecosystems. Techniques such as controlled burns and invasive species management promote biodiversity and resilience. They help mitigate the release of stored carbon through soil erosion and habitat loss.
Managing Forest Resources
Managing forest resources responsibly means understanding the balance between utilization and conservation. Practices such as continuous cover forestry can maintain canopy cover while harvesting. This approach supports both carbon sequestration and the health of ecosystems.
Sustainable Forest Management
Sustainable forest management encompasses strategies that ensure forests remain viable for future generations while minimizing carbon emissions.
Certification Standards
Various certification standards exist to guide sustainable practices. Organizations such as the Forest Stewardship Council (FSC) provide frameworks to ensure that the timber industry adheres to ecological and social standards. Certification can also enhance the marketability of wood products by assuring consumers of their sustainability.
Economic Benefits of Sustainability
Investing in sustainable forestry practices can yield significant economic benefits. Financial incentives for maintaining forest health, such as carbon credits, can encourage landowners to prioritize conservation. Furthermore, sustainable forestry practices can lead to increased tourism and recreation opportunities, thus diversifying forest-based economies.
Sustainability in forestry is not only about preserving nature; it’s about securing economic futures and fostering community resilience.
Intro to Carbon Storage in the Atmosphere
Carbon storage in the atmosphere is a critical element of our planet's climate regulation system. Understanding how carbon is stored helps us grasp the wider implications for both ecosystems and human survival. It is essential to recognize that atmospheric carbon contributes significantly to global warming and climate change. This knowledge is vital for forestry professionals and academics invested in sustainable practices and environmental stewardship.
Carbon exists in various forms within the atmosphere, notably carbon dioxide and methane. Carbon dioxide is widely regarded as a primary greenhouse gas, absorbing heat and trapping it within the Earth’s atmosphere. On the other hand, methane, although less abundant, is much more effective at retaining heat. Together, these gases create a delicate balance, which, when disrupted, leads to severe environmental consequences.
The processes through which carbon is stored are multifaceted. They include natural mechanisms like carbon sequestration, where carbon is absorbed by trees and soil, as well as human-induced activities such as land use changes and deforestation. The implications of understanding these processes are profound. By comprehending the source and sink dynamics of carbon, we can formulate effective strategies for mitigation and adaptation against climate change.
Importance of Carbon Storage
- Climate Regulation: Carbon storage serves as a natural regulator of climate. Understanding it can inform conservation strategies and policy decisions.
- Ecosystem Health: Healthy forests and wetlands enhance carbon storage, and their preservation is crucial for maintaining biodiversity.
- Mitigation Strategies: Knowledge of carbon dynamics can aid in developing innovative approaches to reduce emissions through better land management.
In summary, the study of carbon storage in the atmosphere offers key insights into the interconnectedness of natural systems and human impacts. As climate change continues to pose serious challenges, this understanding becomes increasingly critical for the future of our planet and the management of its resources.
"The first step towards alleviating climate change is understanding the role of carbon in our atmosphere. Knowledge drives informed decisions."
To deepen this understanding, further investigation into the roles of carbon dioxide, methane, and their sources will be discussed in subsequent sections.
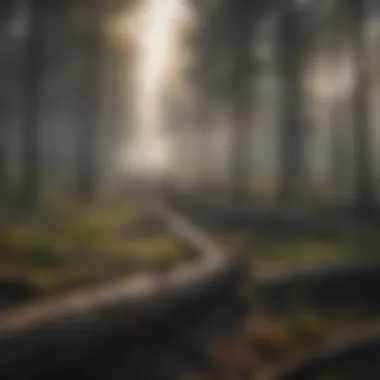
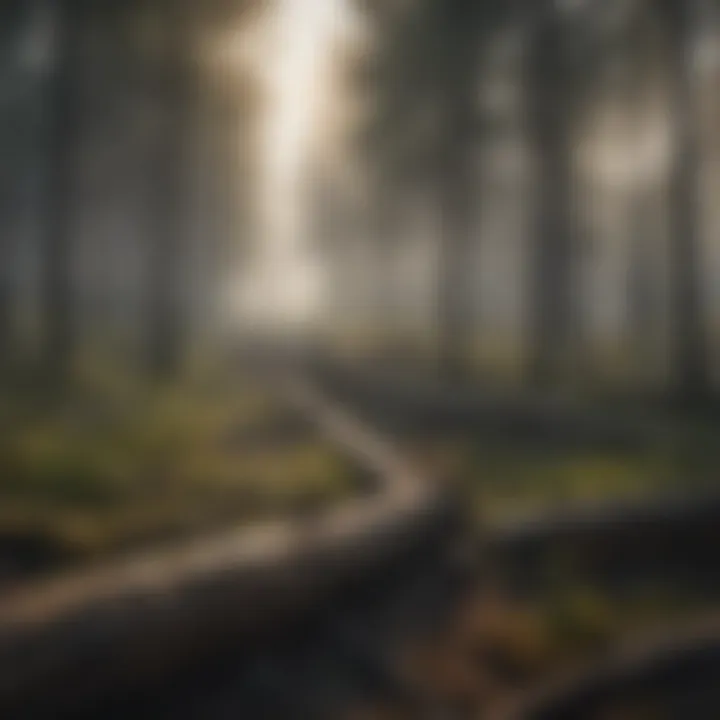
The Role of Carbon Dioxide
Carbon dioxide (CO2) is a fundamental component in Earth's atmospheric chemistry and is pivotal in discussions surrounding climate dynamics. As a greenhouse gas, its presence directly influences temperature regulation on the planet. When CO2 accumulates in the atmosphere, it creates a blanket effect, trapping heat and contributing to global warming. Thus, understanding its role is essential for anyone concerned with environmental science, particularly in forestry, where trees and ecosystems are impacted by changes in climate.
CO2 is not just a marker of industrial impact; it is also a part of natural processes. Photosynthesis is a crucial example where trees absorb carbon dioxide, assisting in growth while releasing oxygen. This relationship underscores the dual nature of carbon—its potential to sustain life and its capability to contribute to climate disturbances. Increased levels of CO2 affect forest health, leading to shifts in species composition, productivity, and resilience against pests and diseases.
Chemical Properties of Carbon Dioxide
Carbon dioxide is a colorless gas with a slightly acidic taste and odor. At room temperature, it exists in a gaseous state and plays a critical role in the carbon cycle. One noteworthy property is its density, which is about 1.5 times that of air. This characteristic enables CO2 to settle in lower layers of the atmosphere, influencing local weather patterns and air quality.
In terms of chemical reactivity, CO2 is non-flammable and does not support combustion. It readily dissolves in water, forming carbonic acid, which can affect soil acidity and nutrient availability. These properties make it significant in ecological studies, especially regarding soil health and forest dynamics.
Sources of Carbon Dioxide Emissions
The emissions of carbon dioxide stem from various sources that can be both natural and anthropogenic. The principal contributors to CO2 emissions include:
- Fossil fuel combustion: This is the largest anthropogenic source, encompassing electricity generation, transportation, and industrial processes.
- Deforestation: Trees are significant carbon sinks. When they are cut down or burned, stored carbon dioxide is released back into the atmosphere.
- Soil respiration: Organic matter decomposition in soils releases CO2, particularly in managed or disturbed ecosystems.
Natural sources also play a role. Volcanic eruptions and oceanic processes emit CO2, but these are typically balanced by natural carbon sinks. In contrast, human activities have tipped this balance, necessitating immediate attention to mitigate impacts.
Effective carbon management strategies are essential for maintaining environmental health and supporting sustainable forestry practices.
Methane and Its Impact
Methane plays a significant role in understanding carbon storage in the atmosphere. As one of the potent greenhouse gases, methane's impact on climate change is increasingly recognized. Its global warming potential is many times that of carbon dioxide over a shorter timeframe. This quality makes a focus on methane essential when examining overall atmospheric carbon dynamics. Understanding its characteristics and sources can inform mitigation strategies, helping to address climate change effectively.
Characteristics of Methane
Methane (CH₄) is a colorless, odorless gas. It is lighter than air and possesses a higher capacity for heat retention than carbon dioxide. In fact, over a 20-year period, methane is estimated to be 84 times more effective at trapping heat in the atmosphere than carbon dioxide. Its molecular structure allows it to absorb infrared radiation effectively, leading to enhanced greenhouse effects.
Another noteworthy characteristic is its biogenic production. Methane is released during the breakdown of organic materials through anaerobic processes, particularly in wetlands. Additionally, methane can be produced through geothermal and thermogenic processes found in natural gas extraction. These complexities in its nature make understanding methane vital for climate modeling and mitigation planning.
Methane Emissions Sources
Methane emissions stem from a variety of sources, both natural and anthropogenic. Here are the primary contributors:
- Agriculture: Livestock digestion and manure management are significant sources. Cattle, for example, produce methane during digestion through enteric fermentation.
- Landfills: Decomposing organic waste in landfills generates methane as a byproduct, especially when oxygen is limited.
- Natural Gas Systems: Leakages during extraction, processing, and distribution of natural gas contribute considerable amounts of methane.
- Wetlands: Natural wetlands release methane as part of their microbial processing of organic material.
- Rice Cultivation: The flooding of rice paddies creates an anaerobic condition conducive to methane production.
By addressing these sources, effective strategies can be put in place to curtail methane emissions, thus addressing one of the critical components of climate change.
“Mitigating methane emissions can yield rapid benefits for climate change and air quality.”
Mechanisms of Carbon Storage
In examining the storage of carbon in the atmosphere, it is essential to delve into the mechanisms that facilitate this process. Understanding these mechanisms helps to comprehend how carbon is managed and released back into the environment. The storage of carbon is not merely a passive process; it includes complex interactions between various elements that contribute to both natural and anthropogenic carbon cycles.
Effective carbon storage mechanisms play a crucial role in regulating atmospheric carbon levels, with implications for climate change and ecosystem health. These mechanisms involve diverse processes that can sequester carbon dioxide and methane, thereby mitigating greenhouse gas effects. Recognizing these mechanisms benefits not only forestry professionals but also researchers and policymakers seeking sustainable environmental strategies.
Carbon Sequestration Processes
Carbon sequestration involves capturing and storing atmospheric carbon dioxide. This can occur naturally through various processes or can be enhanced through human intervention. Key natural sequestration processes include the absorption of carbon dioxide by oceans and terrestrial ecosystems.
- Physical Sequestration: Ocean waters absorb carbon dioxide from the atmosphere. This process reduces the gas's presence in the air, limiting its greenhouse effect.
- Biological Sequestration: Plants and trees absorb carbon dioxide during photosynthesis, converting it into biomass. This transformation helps to create a natural reservoir of carbon.
- Chemical Sequestration: Involves specific reactions where carbon dioxide combines with minerals to form stable compounds, which can be stored safely in the earth.
These processes are essential for regulating the carbon cycle and play a role in mitigating climate change effects. They also emphasize the importance of maintaining healthy ecosystems and promoting biodiversity.
Soil's Role in Carbon Storage
Soil functions as a significant carbon sink, storing organic carbon produced from decaying plant and animal matter. The soil's ability to sequester carbon depends on various factors, including its composition and management practices. Healthy soils can store substantial amounts of carbon, which in turn influences both environmental and agricultural productivity.
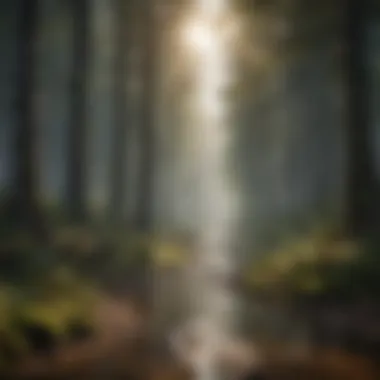
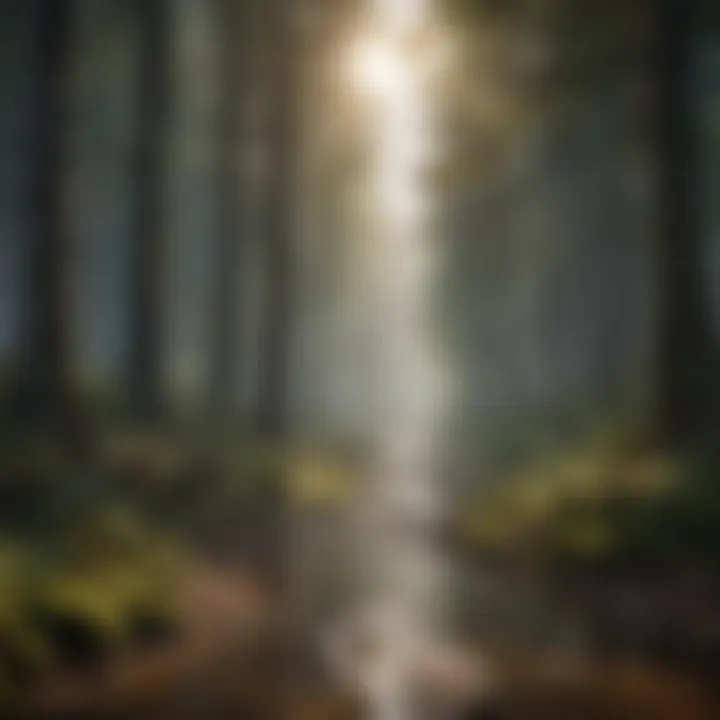
- Soil Composition: Different types of soil have varying capacities for carbon storage. For example, clay-rich soils tend to sequester more carbon than sandy soils.
- Soil Management: Agricultural practices significantly impact soil carbon levels. Techniques such as no-tillage, cover cropping, and organic farming can enhance carbon sequestration.
Soil serves not only as a carbon reservoir but also supports plant growth, playing a fundamental role in the broader carbon cycle.
Forests as Carbon Sinks
Forests are recognized as one of the most effective natural carbon sinks on the planet. They play a vital role in sequestering carbon dioxide through photosynthesis, storing carbon in biomass and soil. The accumulation of carbon in forests is critical for maintaining balanced ecosystems and combating climate change.
- Biodiversity: The diversity within forest ecosystems contributes to their carbon storage capacity. Different species of trees and plants have varying efficiencies in capturing and storing carbon.
- Forest Management: Sustainable forest management practices enhance carbon sequestration. Techniques such as reforestation and afforestation can increase the carbon storage capacity of forested areas.
Through understanding the dynamics of forest ecosystems, we gain insight into how to maintain and enhance their role as carbon sinks, which is crucial in the global fight against climate change.
Carbon Cycle Dynamics
Natural Carbon Cycle
The natural carbon cycle refers to the continuous movement of carbon among the air, water, soil, plants, and animals. It involves several key processes: photosynthesis, respiration, decomposition, and ocean uptake. In photosynthesis, plants absorb carbon dioxide from the atmosphere and convert it into organic matter. This organic matter becomes food for herbivores and subsequently for higher trophic levels. During respiration, both plants and animals release carbon dioxide back into the atmosphere, completing one aspect of the cycle.
Decomposition is another vital aspect. When organisms die, decomposers break down their bodies, returning carbon to the soil or releasing it into the atmosphere. Furthermore, oceans play a critical role by absorbing significant amounts of carbon dioxide, regulating atmospheric levels and influencing climate.
"Understanding the natural balance of the carbon cycle is essential for effective climate action and long-term ecological health."
Human Impacts on the Carbon Cycle
Human activities have profoundly impacted the natural carbon cycle, leading to increased levels of carbon dioxide and methane in the atmosphere. The combustion of fossil fuels, land-use changes, and deforestation are primary contributors to this imbalance. When forests are cleared for agriculture or urban development, the carbon stored in trees is released back into the atmosphere, exacerbating greenhouse gas concentrations.
Moreover, the industrial processes provide large emissions of carbon dioxide, significantly altering the balance within the cycle. Agriculture also contributes through practices such as tillage, which disturbs soil carbon, and livestock production, which releases methane. The enhanced greenhouse effect leads to climate change, further complicating carbon dynamics.
Recognizing these anthropogenic influences highlights the importance of developing sustainable practices to mitigate their negative effects on the carbon cycle.
In summary, carbon cycle dynamics encompass both the natural mechanisms sustaining life on Earth and the profound effects of human activity disrupting these systems. A thorough understanding can guide efforts to enhance carbon storage and regulate atmospheric levels of greenhouse gases.
Atmospheric Interactions and Feedback Loops
Atmospheric interactions and feedback loops are crucial components in understanding how carbon is stored and behaves within the atmosphere. These dynamics affect not only carbon concentrations but also broader climate patterns. The interactions between different atmospheric components can either enhance or mitigate the effects of greenhouse gases. A comprehensive understanding of these interactions is necessary, especially for forestry professionals and academics involved in environmental science.
- Role of Water Vapor
Water vapor plays a significant role in the Earth’s climate system. As the most abundant greenhouse gas, it amplifies the warming effects of carbon dioxide and methane. When the atmosphere warms, it can hold more moisture, leading to an increase in water vapor. This process creates a feedback loop:The implications of this feedback loop are significant. It means that even small changes in carbon dioxide levels can lead to larger increases in global temperatures. These dynamics can change precipitation patterns, diluting local ecosystems and affecting forest health. Understanding this relationship is essential for creating effective strategies to combat climate change and mitigate its impacts on carbon storage in the atmosphere. - Feedback Mechanisms Involving Carbon
Feedback mechanisms related to carbon are critical to grasping the full scope of carbon dynamics in the atmosphere. There are various ways in which these mechanisms operate:These feedback mechanisms illustrate the interconnectedness of systems. The changing climate does not only see an increase in carbon dioxide but also produces a series of reactions that compound the situation. Each mechanism can elevate the challenges in managing atmospheric carbon and demonstrate the importance of a holistic view in climate strategy.
- Higher temperatures increase evaporation, which adds more water vapor to the atmosphere.
- Increased water vapor enhances warming, leading to even higher temperatures.
- Carbon Cycle Feedbacks: Processes such as permafrost thawing release stored carbon, contributing to atmospheric carbon increase. This, in turn, can elevate global temperatures, leading to further permafrost melting.
- Oceanic Feedbacks: The oceans absorb a significant amount of carbon dioxide. As ocean temperatures rise, their ability to hold carbon decreases, leading to higher concentrations of carbon dioxide in the atmosphere.
- Vegetative Feedbacks: Changes in vegetation due to climate effects can alter carbon uptake rates. For instance, forest die-offs from drought or disease can release carbon, disrupting local carbon balances.
Understanding atmospheric interactions and feedback loops illuminates the complex dance between carbon, temperature, and climate systems and highlights the urgency for immediate action.
In summary, both water vapor and carbon feedback mechanisms are integral to the larger narrative of carbon dynamics in the atmosphere. They underscore the necessity for focused research on how these interactions influence climate change. For forestry professionals and academics, recognizing these complexities allows for informed decision-making in management practices and policy development.
Historical Context of Carbon in the Atmosphere
The historical context of carbon in the atmosphere is essential in understanding the present state of our climate. By examining past emissions trends and the impacts of industrialization, we can better appreciate the complex interactions between human activities and natural carbon storage processes. This context lays the groundwork for developing effective mitigation strategies to combat climate change and enhance carbon sequestration.
Historical Emissions Trends
In analyzing historical emissions trends, it is crucial to consider the significant increase in atmospheric carbon dioxide and methane over the past century. Carbon dioxide levels remained relatively stable until the late 18th century, coinciding with the onset of the Industrial Revolution. Since then, the concentration of carbon dioxide has risen from approximately 280 parts per million (ppm) to over 410 ppm today. This change is primarily due to the combustion of fossil fuels, deforestation, and other anthropogenic activities.
Similarly, methane emissions have seen a marked increase. Methane concentrations in the atmosphere have more than doubled since pre-industrial times. Agriculture, particularly livestock production, and the extraction of fossil fuels contribute significantly to these rising methane levels. The historical record underlines how these emissions trends are not just statistical anomalies but critical indicators of human influence on the atmosphere.
"Carbon emissions from human activities have fundamentally altered the atmospheric composition and are driving climate change at an unprecedented pace."
Impacts of Industrialization
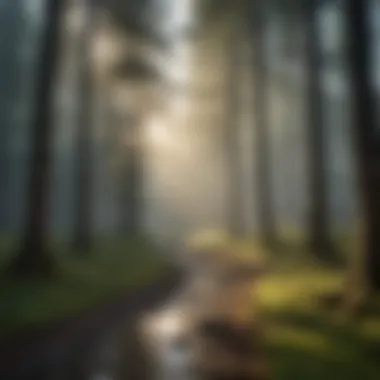
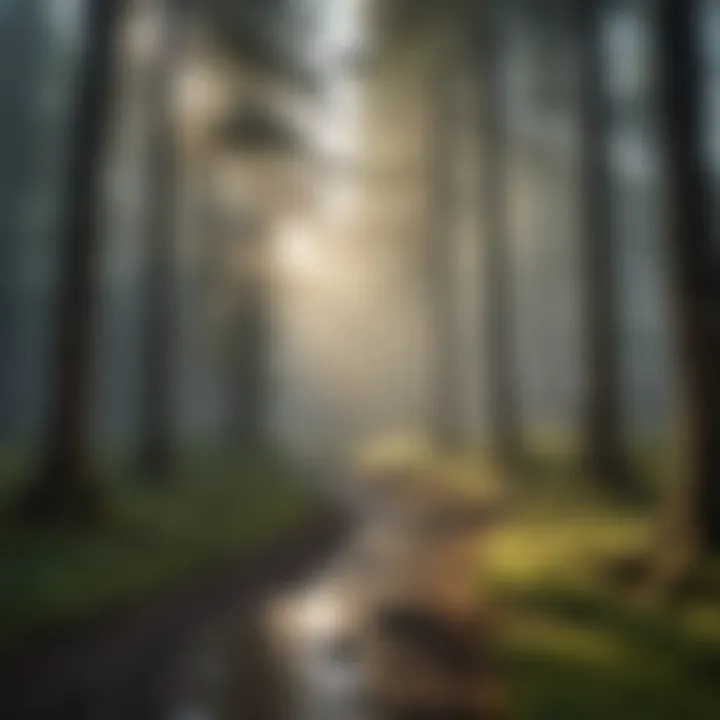
The impacts of industrialization on carbon storage in the atmosphere are profound. Industrial activities have transformed landscapes and ecosystems, disrupting natural carbon sinks such as forests and wetlands. The reliance on fossil fuels for energy has created a persistent carbon load in the atmosphere, leading to an imbalance in the global carbon cycle.
Key points regarding the impact include:
- Deforestation: The clearing of forests for timber and agriculture has led to a decrease in the number of trees available to sequester carbon, thereby increasing atmospheric levels.
- Urbanization: The growth of urban areas has resulted in increased carbon footprints through transportation, energy use, and waste generation.
- Industrial Processes: Manufacturing processes often release significant amounts of greenhouse gases, compounding the overall emissions problem.
Understanding these impacts is vital for forestry professionals and academics. It enables them to devise strategies to restore ecosystems and enhance carbon sequestration effectively. Additionally, acknowledging the historical context helps inform policy decisions aimed at reducing present and future emissions.
Mitigation Strategies
Mitigation strategies are vital in the effort to manage and reduce carbon emissions in the atmosphere. These strategies play a crucial role in addressing the impacts of climate change and maintaining ecological balance. The focus on mitigation acknowledges that current levels of carbon dioxide and methane in the atmosphere exceed pre-industrial figures and are contributing to the warming of our planet. Effective strategies not only target reduction of emissions but also enhance the natural processes involved in carbon sequestration. Understanding these strategies is essential for forestry professionals and academic circles alike, as they offer practical pathways toward sustainability.
Enhancing Carbon Sequestration
Enhancing carbon sequestration is one of the primary mitigation strategies. This involves increasing the amount of carbon stored in various natural systems, notably forests, wetlands, and soils. Here are several approaches that can be utilized:
- Afforestation and Reforestation: Planting new forests and restoring degraded ones can significantly increase the amount of carbon sequestered. Trees absorb carbon dioxide through photosynthesis and store it as biomass.
- Soil Management Techniques: Practices like no-till agriculture, cover cropping, and crop rotation can improve soil health and increase its carbon storage capacity. Healthy soils hold more organic matter and therefore sequester more carbon.
- Wetland Restoration: Wetlands are highly efficient at capturing carbon. Restoring these ecosystems helps improve their capacity to absorb carbon dioxide while also providing vital habitat for wildlife.
Implementing these strategies can lead to both environmental benefits and economic advantages. Monitoring and assessing the potential impacts is important in order to ensure the effectiveness of such initiatives and to communicate the benefits clearly to stakeholders.
Policy Approaches and Regulations
Policy approaches and regulations are essential for implementing effective mitigation strategies. Governments and organizations need a framework to guide actions and determine funding allocations.
- Carbon Pricing: Implementing carbon taxes or cap-and-trade systems creates an economic incentive to reduce emissions. This allows market mechanisms to drive investment toward greener technologies and practices.
- Subsidies for Renewable Energy: Providing financial assistance for renewable energy projects helps to decrease reliance on fossil fuels. Polices promoting solar, wind, and hydro energy can significantly reduce negative environmental impacts.
- Support for Research and Development: Investing in innovative technologies, such as carbon capture and storage, can offer new solutions for minimizing atmospheric carbon levels. More research can lead to enhanced understanding and technology that promotes carbon sequestration.
These policy approaches require collaboration among governments, businesses, and communities. An engaged and informed public can drive support for these initiatives, ultimately enhancing their effectiveness in combating climate change.
Future Directions for Carbon Storage Research
The significance of advancing research on carbon storage cannot be overstated. As the climate crisis intensifies, understanding all dimensions of carbon dynamics becomes critical. Future directions in this field revolve around enhancing efficiency in carbon storage methods, which directly influences how we tackle greenhouse gas emissions. This section will outline key emerging technologies and the necessity of community involvement in carbon management strategies.
Emerging Technologies
New technologies are reshaping the landscape of carbon storage. Innovations like bioenergy with carbon capture and storage (BECCS) presents promising potential. BECCS utilizes biomass to generate energy, then captures and stores the emitted carbon dioxide underground. This method not only provides an energy source but also reduces atmospheric carbon levels.
Moreover, direct air capture (DAC) systems are gaining traction. These facilities pull carbon directly from the atmosphere and either store it underground or use it in products. Such technology has potential to become invaluable as it targets existing atmospheric carbon rather than focusing solely on emissions reductions.
In addition, soil carbon sequestration techniques are evolving, emphasizing agricultural practices that enhance soil organic matter. Techniques like no-till farming and crop rotation contribute to increased carbon storage in soils, benefiting agricultural yields while also mitigating climate impacts.
Key Technologies to Watch:
- Bioenergy with Carbon Capture and Storage (BECCS)
- Direct Air Capture (DAC)
- Enhanced soil carbon sequestration techniques
The Role of Community Engagement
Community engagement plays a pivotal role in the success of carbon storage initiatives. People at the local level are crucial stakeholders when it comes to implementing and supporting mitigation strategies. Effective community involvement can foster stronger relationships between scientists, policymakers, and the public.
Initiatives to educate communities about carbon cycles and storage methods can significantly boost participation in carbon management activities. Communities can contribute to carbon sequestration through practices such as reforestation and sustainable land use. When individuals understand the impact of their actions, they are more likely to engage in environmentally beneficial practices.
"Community-led initiatives enhance the sustainability of carbon storage projects, as they align local interests with broader environmental goals."
Ends
In concluding our exploration of carbon storage in the atmosphere, it is essential to recognize the multi-faceted nature of the topic. Carbon storage is not merely an academic concern; it is a critical component of environmental health and stability. The article highlights the interplay between natural processes and human activities that influence carbon dynamics. Understanding these factors is crucial for developing effective mitigation strategies against climate change.
The significance of carbon dioxide and methane as greenhouse gases cannot be understated. Their impact on global warming poses challenges that necessitate concerted efforts in research and action. Besides, the roles of forests and soils in carbon sequestration illustrate opportunities for enhancing carbon storage through sustainable practices. It is vital for forestry professionals and academics to be aware of these methods to promote healthier ecosystems.
Key considerations that emerge from this discourse include:
- The urgent need for deeper understanding of anthropogenic impacts on the carbon cycle.
- The effectiveness of policies aimed at promoting carbon sequestration techniques.
- The implications of emerging technologies which can change the landscape of carbon storage research.
Furthermore, community engagement is a theme worth stressing. Involving local communities in carbon management will bolster efforts in combating climate change. Their participation can lead to more effective and culturally relevant strategies.
"The path forward must include a blend of scientific research, technological innovation, and active participation from all sectors of society."
This multidisciplinary approach is not just beneficial but necessary for tackling the complex issues surrounding atmospheric carbon. Encouraging collaboration across disciplines can lead to innovative solutions that adequately address the pressing needs of our environment. As understanding deepens, so too does our capacity to enact change, ensuring that future generations inherit a planet capable of sustaining life. The importance of this topic extends far beyond the academic realm; it has real-world implications that resonate throughout ecosystems and communities.