Innovative Strategies for Effective CO2 Storage
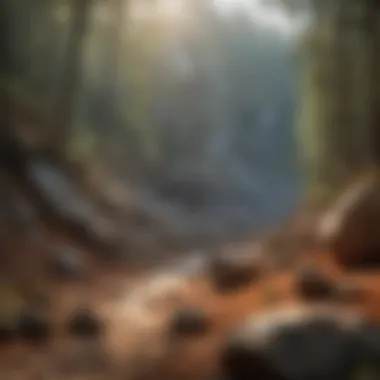
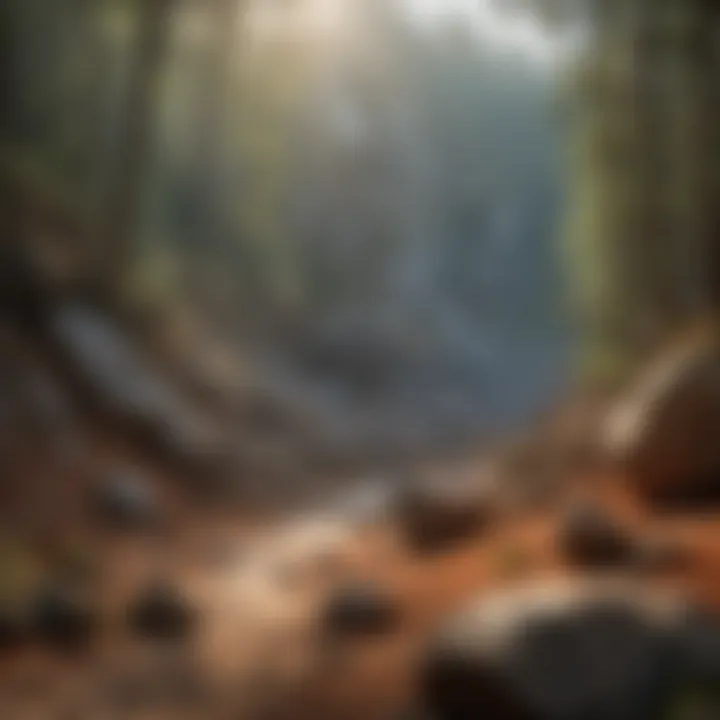
Intro
Carbon dioxide storage is a crucial component in the fight against climate change. Understanding various methods and technologies is imperative for developing effective strategies that can mitigate the adverse effects of rising carbon levels in the atmosphere. This discussion not only touches on technological innovations but also considers natural approaches that have long been utilized in forestry practices.
The integration of these strategies into forestry provides numerous benefits. For instance, forests act as carbon sinks, absorbing significant amounts of carbon dioxide during their growth processes. Thus, forestry practices that focus on maintaining healthy forests can play an even bigger role in carbon storage, making it essential for professionals in this field to remain informed about the latest strategies and technologies.
Understanding Forestry Practices
Forestry practices encompass a range of methods aimed at managing forest resources sustainably. These practices can be broadly categorized into natural and engineered strategies, each with its own unique benefits and challenges. By understanding these categories, professionals can better assess their relevance in the context of carbon dioxide storage.
Types of Forestry Practices
- Natural Forestry: This involves allowing forests to regenerate and evolve in their own way without significant human intervention. Practices such as selective logging and shelterwood cutting help to preserve the forest's biodiversity while still allowing for resource utilization.
- Engineered Forestry: This is characterized by deliberate interventions to improve forest growth and carbon sequestration potential. Techniques in this category might include reforestation and afforestation, where trees are planted in deforested or previously non-forested areas to absorb carbon.
- Agroforestry: This innovative method combines agriculture and forestry practices for enhanced productivity. By integrating trees with crops or livestock, agroforestry systems can significantly enhance carbon storage while also benefiting farmers economically.
Historical Context
Historically, forestry practices have undergone significant evolution. In the past, most forests were managed for immediate economic gain, leading to unsustainable harvesting practices. However, the increasing awareness of environmental issues has shifted focus toward sustainable methods. In modern times, the emphasis is on balancing economic needs with ecological preservation, crucially recognizing the role of forests in climate regulation.
"The sustainable management of forests not only enhances biodiversity but also provides a vital ecosystem service essential for mitigating climate change."
By taking a historical perspective, forestry professionals can glean insights that inform current and future practices. Lessons learned from past mismanagement highlight the importance of responsible stewardship, especially regarding carbon storage strategies.
Principles of Woodland Stewardship
Woodland stewardship is about managing forest ecosystems effectively. This involves employing practices that ensure the health and sustainability of forests.
Conservation Techniques
Conservation techniques are integral to woodland stewardship. These methods include:
- Controlled burns: These help reduce underbrush, promote growth, and enhance carbon sequestration.
- Invasive species management: Removing non-native species can improve biodiversity and forest health, aiding carbon storage capabilities.
- Wildlife habitat protection: Maintaining habitats supports ecological balance and protects the biodiversity necessary for resilient forest ecosystems.
Managing Forest Resources
Effective management of forest resources involves identifying and implementing practices that optimize both growth and carbon absorption. Key aspects include monitoring tree health, employing sustainable harvesting methods, and using technology for better resource assessment. This multifaceted approach ensures that forestry professionals can adapt their strategies based on changing environmental conditions while maximizing carbon sequestration.
Sustainable Forest Management
Sustainable forest management is a holistic approach that takes into consideration environmental, social, and economic dimensions. The goal is to meet the needs of today without compromising the ability of future generations to meet theirs.
Certification Standards
Organizations like the Forest Stewardship Council play a significant role in establishing certification standards that promote sustainable practices. These standards ensure that forests are managed responsibly, supporting both ecological integrity and local economies.
Economic Benefits of Sustainability
Investing in sustainable forest management offers substantial economic benefits. Healthier forests can lead to increased yields of timber and non-timber products. In addition, the adoption of sustainable practices can attract funding from organizations focused on environmental protection, thus providing additional financial support for forestry projects.
Through careful consideration and application of these strategies, forestry professionals can lead the way in effective carbon dioxide storage, reinforcing the critical role of forests in fighting climate change.
Prologue to Carbon Dioxide Storage
Carbon dioxide storage is a crucial component in the global effort to mitigate climate change. With rising CO2 levels, stemming from industrial activities, transportation, and deforestation, the urgency for effective carbon management cannot be overstated. This section introduces the significance of carbon dioxide storage as a mechanism for reducing greenhouse gas emissions and fostering a sustainable environment.
The Importance of Carbon Dioxide Management
Effective management of carbon dioxide plays a critical role in combating climate change. As a greenhouse gas, CO2 traps heat in the atmosphere, contributing to global warming. The consequences of uncontrolled emissions are profound, leading to climate-related disasters, biodiversity loss, and adverse impacts on human health. Thus, prioritizing carbon management can help stabilize the climate by
- reducing atmospheric concentrations of CO2,
- enabling the achievement of international climate agreements, and
- fostering a transition towards sustainable energy systems.
The integration of carbon storage solutions is an essential strategy in supporting these management efforts. It also offers potential economic benefits through the development of new technologies and job creation in related sectors.
Overview of Storage Methods
There are various methods for carbon dioxide storage, each coming with distinct features and implications. Broadly, these methods can be categorized into natural and engineered processes.
- Natural Storage Methods:
These include carbon sinks such as forests, soils, and wetlands, which naturally absorb and store carbon. The preservation and enhancement of these ecosystems can improve biodiversity while effectively sequestering CO2 over long periods. - Engineered Storage Technologies:
These involve technological solutions, such as Carbon Capture and Storage (CCS), where CO2 emissions from industrial sources are captured before they are released into the atmosphere. The captured CO2 can then be injected into geological formations deep underground, storing it safely away from the climate system. - Utilization of Captured Carbon:
This method involves using captured CO2 for commercial products or processes, such as carbonated beverages, plastics, or biofuels. Such an approach not only reduces the net emissions but also contributes to a circular economy.
Each method presents unique advantages and challenges that need careful consideration in context of their long-term viability and environmental impact. Understanding these options is essential for informed policy-making and effective climate action.
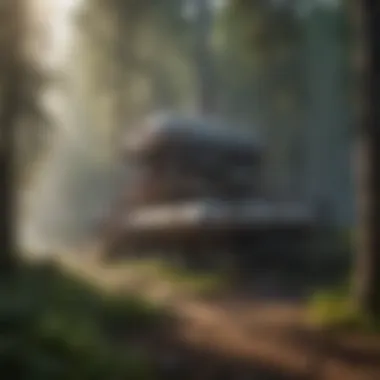
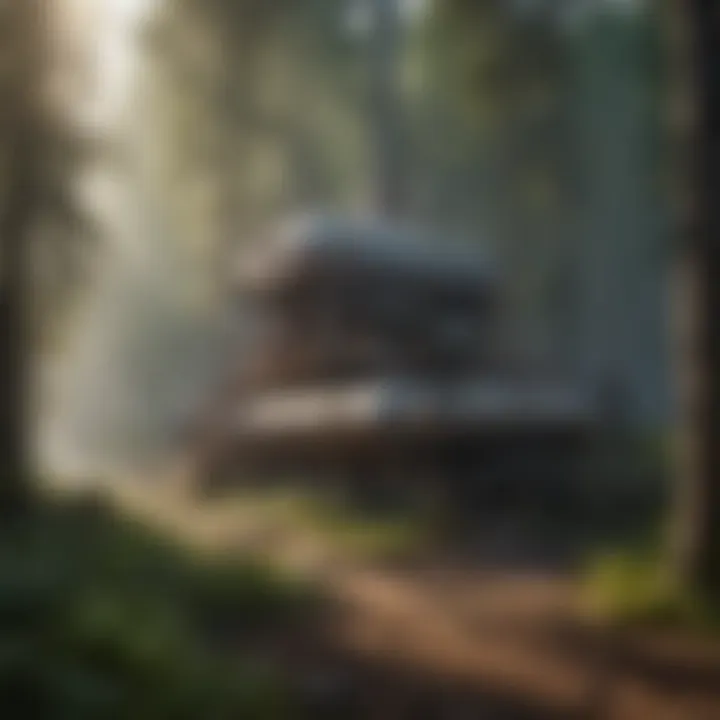
Natural Carbon Storage Solutions
Natural carbon storage solutions involve processes that naturally sequester carbon dioxide from the atmosphere. These processes are crucial in mitigating climate change due to their ability to effectively trap carbon in various ecosystems. By leveraging naturally occurring systems, we can enhance the capacity for carbon storage, benefiting both the environment and biodiversity.
Forests as Carbon Sinks
Forests play a significant role in carbon storage. They absorb carbon dioxide during photosynthesis and store it in their biomass, roots, and soil. Trees can live for decades, and some species can sequester thousands of tons of carbon over their lifetimes. The effectiveness of forests as carbon sinks varies based on location, forest type, and forest management practices.
Positive benefits of forests as carbon sinks include:
- Biodiversity Support: Forests are home to many species, thus promoting biodiversity.
- Soil Health: Healthy forest ecosystems improve soil structure and fertility, leading to better agricultural yield.
- Water Regulation: Forests help in maintaining water cycles, which is imperative for local climates.
However, forests face threats from deforestation and climate change itself. Protecting existing forests and reforesting degraded areas are critical strategies to enhance their capacity for carbon sequestration.
Soil Carbon Sequestration
Soil carbon sequestration involves storing carbon in soil organic matter. This process is essential for maintaining soil health and improving agricultural productivity. Healthy soils can significantly increase their carbon content through the addition of organic materials like compost or cover crops.
The key advantages of soil carbon sequestration include:
- Improved Soil Structure: Enhances aeration and water retention, which supports plant growth.
- Increased Nutrient Availability: Organic matter enriches soils, benefiting crops.
- Mitigation of Erosion: Well-structured soil is less prone to erosion.
Adopting agricultural practices such as no-till farming or agroforestry can promote soil carbon storage. The challenge remains in measuring and verifying carbon levels in soils over time, which is crucial for assessing the efficacy of these practices.
Wetlands and Their Role
Wetlands act as vital carbon reservoirs. They accumulate organic matter due to stagnant water that slows decomposition, leading to higher carbon storage concentrations. The primary types of wetlands include marshes, swamps, and bogs, each contributing differently to carbon sequestration.
Benefits of wetlands in carbon dioxide storage are:
- Water Quality Improvement: Wetlands filter pollutants, providing cleaner water sources.
- Biodiversity Habitat: They support diverse plant and animal life, making them ecologically rich.
- Flood Protection: Wetlands absorb excess rainwater, mitigating flooding effects.
However, wetlands are at risk due to drainage for agriculture and urban development. Protecting and restoring these areas are vital for both carbon storage and ecosystem health.
"Natural carbon storage solutions offer diverse benefits that extend beyond carbon capture. They enhance ecosystem resilience and promote biodiversity, addressing environmental issues alongside climate change."
Engineered Carbon Dioxide Storage Technologies
Engineered carbon dioxide storage technologies represent a critical component in the global strategy to combat climate change. They offer a methodological approach to sequester carbon dioxide from the atmosphere or at the point of emission. By implementing these advanced systems, we can significantly reduce greenhouse gas concentrations. This section discusses pivotal technologies including Direct Air Capture Systems, Carbon Capture and Storage (CCS), and the utilization of captured carbon. Each technology carries unique advantages and considerations.
Direct Air Capture Systems
Direct Air Capture (DAC) systems are engineered to remove carbon dioxide directly from the ambient air. This process typically involves large fans that pull air into a chemical solution or solid sorbent material capable of binding CO2. Once captured, the carbon dioxide can be stored underground or utilized for other applications.
The significance of DAC is found in its ability to address historic emissions, thus reversing some damage already caused.
Some key points regarding DAC include:
- Scalability: These systems can be sized to meet different operational needs.
- Energy Requirements: They require significant amounts of energy, which often raises questions about their net carbon reduction impact unless powered by renewable sources.
- Cost: Current estimates suggest that DAC is expensive compared to more traditional carbon capture methods, and as such, investment in research and development is needed.
Carbon Capture and Storage (CCS)
Carbon Capture and Storage (CCS) technology is widely implemented in various industrial processes, especially in fossil fuel power plants. This method captures carbon dioxide emissions at their source before they reach the atmosphere, compresses the CO2, and transports it to a storage site. Typical storage sites include depleted oil and gas fields or saline aquifers.
The benefits of CCS are as follows:
- Immediate Impact: It allows the continued operation of fossil fuel infrastructure while minimizing environmental impact.
- Mature Technology: CCS has been deployed in several locations worldwide, providing valuable data and experience.
- Economic Incentives: By utilizing captured CO2 for enhanced oil recovery, companies can improve the feasibility of CCS projects.
However, challenges remain:
- Storage Risk: There are concerns regarding the long-term integrity of CO2 storage sites.
- Public Perception: Acceptance among local communities can vary based on risk concerns and public education.
Utilization of Captured Carbon
Captured carbon offers various pathways for utilization, transforming it from a harmful pollutant into a viable resource. This concept is often referred to as carbon capture, utilization, and storage (CCUS). Utilization can take on several forms:
- Building Materials: Carbon dioxide can be converted into carbonates for use in cement production or as a feedstock for synthetic fuels.
- Chemical Products: It can also serve as a raw material for creating chemicals such as methanol or urea, thus reducing reliance on fossil fuels.
- Food and Beverage Industry: There are applications in the food and beverage sectors, particularly in carbonating drinks.
Utilizing captured carbon not only mitigates overall emissions but also stimulates economic growth through new markets and technologies.

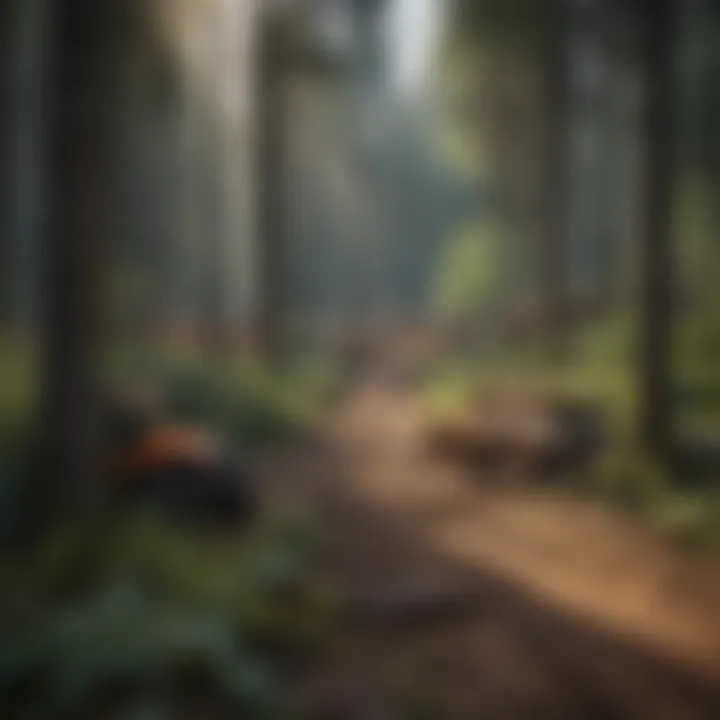
"The future of carbon management will depend heavily on our ability to innovate and adapt technologies for carbon capture and storage effectively."
The promise of engineered carbon dioxide storage technologies offers hope. Yet, they must be integrated thoughtfully into the broader efforts to transition to a sustainable low-carbon economy.
Bioenergy with Carbon Capture and Storage (BECCS)
Bioenergy with Carbon Capture and Storage combines energy production from biomass with the capture and storage of carbon dioxide emissions. This method presents a compelling possibility for reducing greenhouse gas emissions while generating energy. In the context of carbon dioxide management, BECCS stands out for its dual role: it not only provides renewable energy but also has the potential to result in negative emissions. By removing CO2 from the atmosphere, BECCS addresses climate change directly, making it a vital strategy in carbon management discussions.
The BECCS Process Explained
The BECCS process involves several steps. First, biomassโsuch as agricultural residues, wood chips, or energy cropsโis cultivated and harvested. Then, this biomass is converted into bioenergy through combustion or biochemical processes. The carbon dioxide produced during this conversion is captured before it can enter the atmosphere. The captured CO2 is subsequently transported to a suitable geological formation for long-term storage.
- Biomass Production: Biomass is sourced sustainably, ensuring minimal environmental impact.
- Energy Conversion: Various methods can be used, such as direct combustion or anaerobic digestion, to generate energy.
- Carbon Capture: Technologies like amine-based absorption are employed to capture CO2 at the emission point.
- Storage: Finally, the captured CO2 is injected underground, often deep into depleted oil and gas fields or saline aquifers.
This process allows for a circular carbon economy, where CO2 is effectively removed from the atmosphere and not released back.
Advantages of BECCS
The BECCS approach offers several significant benefits:
- Climate Mitigation: By capturing more CO2 than emitted, BECCS can contribute to negative emissions, helping to mitigate climate change.
- Renewable Energy Generation: It provides a source of renewable energy, reducing dependence on fossil fuels.
- Sustainable Development: The growth of biomass can promote agricultural development and create new economic opportunities.
"BECCS offers a unique blend of climate action and energy security, marking a significant shift in our approach to sustainable energy."
Challenges and Limitations
Despite the clear advantages, BECCS also faces several challenges:
- Resource Competition: Biomass cultivation may compete with food production, leading to food security concerns.
- High Costs: The technology for carbon capture can be expensive, impacting the overall economic feasibility of BECCS.
- Storage Risks: Ensuring the long-term safety of CO2 storage sites presents technical and regulatory hurdles.
Measurement and Monitoring of Carbon Sequestration
The accurate measurement and monitoring of carbon sequestration is critical in evaluating the efficacy of carbon dioxide storage strategies. It allows researchers and policymakers to assess how well different methods are performing in terms of capturing and retaining carbon dioxide. This section focuses on the various techniques employed for measuring carbon storage and the long-term practices necessary for effective monitoring. Understanding these elements enhances the reliability and transparency of carbon storage initiatives.
Techniques for Measuring Carbon Storage
There are several established techniques for measuring carbon storage. Each method has its own strengths and weaknesses, which are important to understand in the context of specific applications:
- Ground-Based Measurements: These include direct sampling and remote sensing technologies to assess vegetation carbon stocks. Ground-based biomass inventory techniques often incorporate tree measurements and soil sampling to estimate carbon density.
- Satellite Observations: Satellite technology provides a broader perspective on land use changes and vegetation cover. Measurements from satellites can inform on the large-scale impacts of land management practices on carbon sequestration.
- Soil Carbon Analysis: This technique involves chemical and physical analysis of soil samples to quantify the amount of carbon stored in the soil. Techniques such as soil coring and the use of spectroscopic methods can provide crucial data.
- Ecosystem Models: Computational models combine various data sources to simulate carbon dynamics over time. These models make predictions about carbon storage based on parameters such as climate, land use change, and human activity.
Utilizing a blend of these techniques is often most effective, as it allows for cross-validation and a more comprehensive understanding of carbon storage metrics.
Long-term Monitoring Practices
Long-term monitoring of carbon sequestration is essential to understand the persistence of carbon stocks over time. This aspect of carbon management involves ongoing assessment to capture changes due to climatic factors, land-use changes, or other environmental influences. Key practices include:
- Regular Sampling and Data Collection: Consistent data collection ensures that trends in carbon storage can be tracked. This includes annual or biannual assessments that collect data on vegetation biomass, soil carbon levels, and more.
- Implementation of Baseline Studies: Establishing a baseline before storage activities commence allows for comparison over time. Understanding initial conditions is crucial in evaluating the impact of any carbon storage initiative.
- Use of Technology: Automation and robotic systems can enhance monitoring capabilities. Technologies such as drones and automated sensors provide real-time data on carbon levels and ecosystem health.
- Community Engagement: Involving local communities in monitoring practices not only fosters transparency but also increases local stewardship of carbon storage practices. Engaged communities can assist in ongoing measurements and share local ecological knowledge.
Continuous and systematic monitoring is crucial for validating carbon storage practices and ensuring effectiveness in mitigating climate change while supporting ecological health.
Effective measurement and long-term monitoring create the necessary framework to assure stakeholders of the carbon storage systems' resilience and reliability. As such they provide critical data helping refine future strategies and policies.
Policy and Regulation in Carbon Storage
Understanding the policy and regulatory landscape is crucial for advancing carbon dioxide storage initiatives. Policies shape the frameworks within which carbon storage can thrive. They can provide incentives for innovation, guide investments, and ensure compliance with environmental standards. Clearly defined regulations will help manage risks associated with the long-term storage of carbon dioxide while aligning with national and international climate goals. The effectiveness of carbon storage strategies largely depends on the legal and policy environment in which they are implemented.
Current Policy Frameworks
Several existing policies play a significant role in promoting carbon storage technologies. Regions around the world have begun to develop comprehensive policies that encourage research and development. These frameworks can include grants, tax incentives, and enforcement of emissions trading systems.
Key elements of current policy frameworks include:
- Nationally Determined Contributions (NDCs): Many countries, as part of their commitments to the Paris Agreement, integrate carbon capture and storage goals into their NDCs.
- Regulatory Guidelines: Effective regulations within jurisdictions must clearly outline the operational standards for carbon storage facilities. This includes site selection, monitoring, and reporting processes.
- Public Awareness Programs: Educating stakeholders and the public about the benefits of carbon storage promotes acceptance and support for these technologies.
Such policies have shown positive impacts. Regions that adopt supportive frameworks can attract private investments, making advancements in carbon storage more feasible. Countries like Norway and the United States have been pioneers, implementing rules and providing financial support for carbon capture and storage projects.
Future Directions in Regulation
Looking ahead, the future of carbon storage regulation will likely evolve in response to emerging technologies and geopolitical shifts. As climate change impacts intensify, regulations must adapt to new scientific understandings and technological advancements.
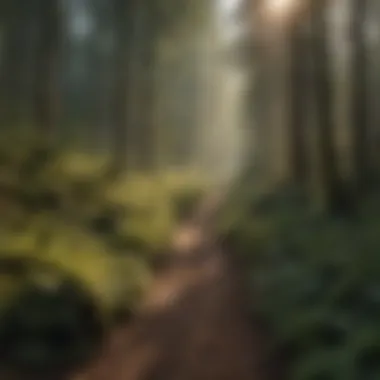
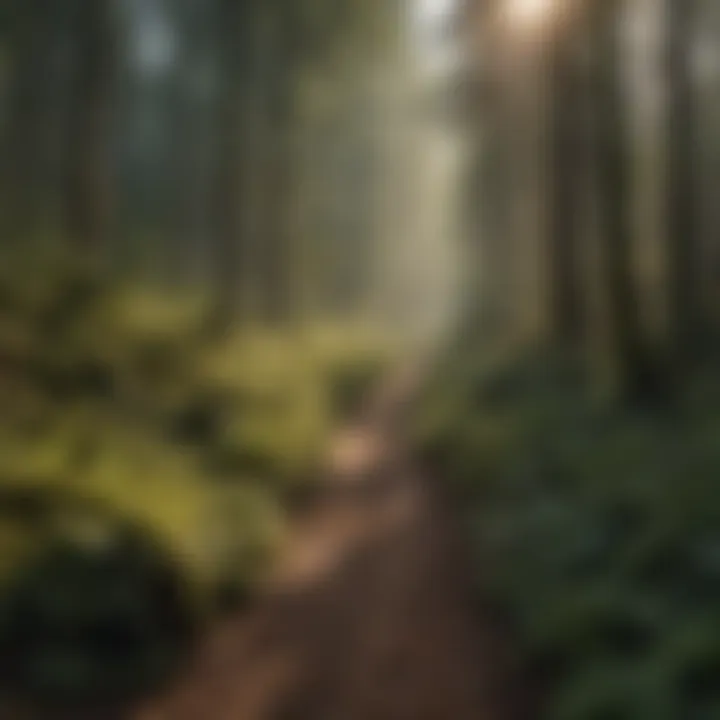
Future regulatory trends may involve:
- Stronger International Collaboration: As carbon pollution knows no borders, future regulations may focus on cross-border cooperation to share best practices and harmonize standards.
- Adaptive Regulatory Policies: With potentially rapid technological development, adaptive regulations will be necessary to balance innovation and safety effectively. This may mean periodic reviews of existing regulations to keep pace with new developments.
- Incorporating Real-Time Data Monitoring: Regulations may embrace technological solutions that allow for continuous monitoring of carbon storage sites, enhancing transparency and safety compliance.
Creating a robust regulatory framework is essential. Policymakers need to be proactive in addressing the challenges of carbon storage. By focusing on sustainable regulations, they can foster an environment that supports innovative carbon storage solutions, ultimately benefiting both the economy and the environment.
"Effective policy frameworks can enable significant strides in carbon dioxide storage, acting as a catalyst for sustainable development and climate resilience."
The ongoing dialogue among various stakeholders, including governments, researchers, and industry players, will be vital in shaping the future regulatory landscape for carbon storage.
Case Studies of Successful Carbon Storage Initiatives
Understanding case studies of successful carbon storage initiatives is crucial for several reasons. First, they offer real-world applications of theoretical concepts and technologies discussed in previous sections. These examples provide insights into the applicability and efficiency of various methods. By analyzing actual experiences, stakeholders can identify best practices, potential pitfalls, and guidelines that can be replicated or avoided in future projects. \n\nSecond, these case studies illuminate the advantages of specific storage techniques. They can showcase how carbon capture and storage (CCS) projects not only reduce greenhouse gas emissions but also support economic development and job creation in local communities. By presenting strong evidence of efficacy, successful initiatives can help build more credible and reliable frameworks for policymakers and investors committed to combating climate change. \n\nLastly, examining these case studies can foster greater collaboration between academia, industry, and government. Stakeholders can learn from shared experiences, leading to improved technology evolution and enhanced strategies for carbon storage worldwide. \n\n### Global Examples of Effective Storage
There are several notable examples of effective carbon dioxide storage initiatives globally. Each of these projects has unique characteristics and demonstrates different technologies.
- Sleipner Project (Norway): Launched in 1996, the Sleipner project involves the capture of CO2 from natural gas processing and its injection into the Utsira Formation, a geological formation located under the North Sea. The project has successfully stored over 1 million tons of CO2 per year. It serves as a pioneering example of offshore geological storage.
- Quest Project (Canada): Quest commenced in 2015 and uses a novel CCS technique to capture CO2 emissions from the refining process at its Canadian oil sands operation. The project captures over 1.1 million tons of CO2 annually and injects it deep underground into a saline aquifer. This initiative has proven to significantly reduce the carbon footprint of fossil fuel extraction.
- Gorgon Project (Australia): This is one of the largest natural gas projects in the world, integrating a CCS component. The project aims to store 3 to 4 million tons of CO2 each year beneath Barrow Island. Despite facing challenges related to implementation, it highlights the complexities and potential of large-scale CO2 storage as part of a broader energy system.
These global examples not only highlight technological effectiveness but also their impact on local and global scales, indicating a path forward for the expansion of carbon storage initiatives.
Lessons Learned from Field Trials
Field trials are vital in drawing conclusions about the effectiveness of carbon storage technologies. They provide essential feedback on methods employed and help in optimizing current practices.
- Need for Monitoring: Successful carbon storage relies heavily on robust monitoring techniques. Continuous tracing and verification of stored CO2 are essential for anticipating potential leaks and ensuring long-term safety.
- Public Acceptance: Engaging stakeholders and addressing community concerns early in the planning stages are crucial. Projects that involve local communities and educate them about potential benefits tend to earn higher levels of public support.
- Adaptation of Techniques: Each geographical location presents unique geological challenges. Flexibility in adapting carbon storage methods to local geologies has proven necessary. Some technologies perform well under specific conditions and may need modifications in different settings.
- Policy Alignment: Understanding and aligning with regulatory frameworks is imperative. Successful case studies demonstrate that having clear, supportive policies can significantly enhance the implementation of storage technologies.
"Lessons from past projects can guide new efforts in carbon storage, ensuring that we build on successes and learn from failures."
The Future of Carbon Dioxide Storage
The future of carbon dioxide storage is increasingly relevant as the world grapples with climate change and strives for sustainable practices. With emissions rising, we need effective storage solutions. Current methods are a starting point, but innovators are finding new ways to make carbon dioxide management more effective. The implications of these advancements are enormous.
Innovative technology, policy updates, and collaborative efforts will be crucial for shaping viable carbon storage strategies. Embracing a mix of ideas can convince societies to consider carbon storage as part of their broader climate policy.
As we explore this topic, itโs essential to recognize several key aspects:
- The growing demand for integrated solutions involving both natural and engineered processes.
- The importance of scaling these solutions to meet the needs of different regions and industries.
- The potential for technological advancements to enhance existing methods, while addressing safety and environmental concerns.
Innovative Approaches on the Horizon
Innovative approaches to carbon dioxide storage are emerging as researchers and industry leaders develop new technologies. Some promising strategies include:
- Enhanced Geological Storage: This method improves upon traditional geological storage by using advanced modeling to select optimal sites. Monitoring technology ensures the integrity of storage sites, helping to mitigate leakage risks.
- Mineral Carbonation: This approach involves reacting carbon dioxide with naturally occurring minerals to form stable carbonates. This not only stores carbon but also creates potential materials for construction and other uses. This method shows great potential for long-term sequestration.
- Biochar Production: Converting biomass into charcoal-like material can sequester carbon. Biochar can be added to soils, improving fertility while locking away carbon. This dual benefit is appealing for agricultural sectors.
Investments in research and development are key to unlock these innovative methods. Collaborative partnerships between governments, academia, and industry will drive progress.
Scaling Up Storage Solutions
Scaling up carbon storage solutions is critical to achieving significant emissions reductions. Key factors must be considered as we move forward:
- Infrastructure Investment: Significant investment in infrastructure is needed for storage sites and transportation systems. Pipelines for delivering captured carbon to storage sites are vital for effective scaling. The expansion of these networks will facilitate widespread adoption of carbon storage techniques.
- Regulatory Frameworks: Strong policies guiding the development and operation of storage sites are essential. Standards must ensure safety and environmental protection while promoting investment.
- Public Engagement: Educating communities about the benefits and safety of carbon storage can help gain public support. This is crucial for the success of large-scale projects.
- Long-term Commitment: Developing carbon storage capabilities is not a short-term effort. A long-term commitment to innovation and deployment across a diverse range of applications will be necessary.
Collaboration across different sectors will yield better solutions for large-scale deployment.
As we look to the future, the integration of all these elements will support the effective scaling of carbon dioxide storage. This will play a pivotal role in combating climate change and ensuring a sustainable environment for future generations.
Finale
The conclusion serves as a critical phase in any exploration of carbon dioxide storage strategies and technologies. It synthesizes the various elements covered throughout the article. Furthermore, it connects the dots between scientific understanding and practical implementation. As greenhouse gas emissions rise, the urgent need for viable carbon management solutions becomes increasingly clear. Understanding these solutions fosters effective discussions regarding policy and sustainable practices.
Summarizing Key Findings
Central to our findings are various methodologies highlighted throughout this article:
- Natural Solutions: Forests, wetlands, and soils play significant roles in sequestering carbon naturally. Their preservation and enhancement is vital.
- Engineered Systems: Technologies like Direct Air Capture and Carbon Capture and Storage (CCS) mark significant advancements in our ability to remove and store CO2.
- Bioenergy with Carbon Capture and Storage (BECCS): This integrates sustainable energy production with carbon reduction, presenting an innovative approach to addressing emissions.
Each of these strategies contributes uniquely, yet they are interlinked. The synergistic relationship among these methods elucidates a multifaceted approach to combat climate change. The article illustrates that while challenges remain, the benefits and advancements in carbon storage technologies provide pathways towards effective climate action.
Implications for Forestry and Environmental Science
The implications of these findings for forestry and environmental science are vast. The role of natural ecosystems as carbon sinks supports not only climate change mitigation but also contributes to biodiversity and soil health.
- The practices that maintain and enhance forest health align with carbon storage goals. Such efforts promote sustainable forestry and biodiversity conservation.
- The importance of soil management cannot be overstated. Healthy soil is a critical component in the effective sequestration of carbon. Practices such as cover cropping and reduced tillage are vital.
- Wetlands also play a crucial role. Conserving and restoring our wetlands can lead to significant carbon storage, while also supporting wildlife and water quality.
By integrating carbon management strategies into forestry, professionals can align environmental health with climate objectives. This understanding leads to informed decision-making about land-use practices and policy development.