Exploring Biochar Production Equipment for Sustainability
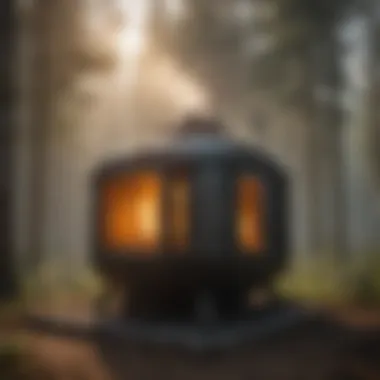
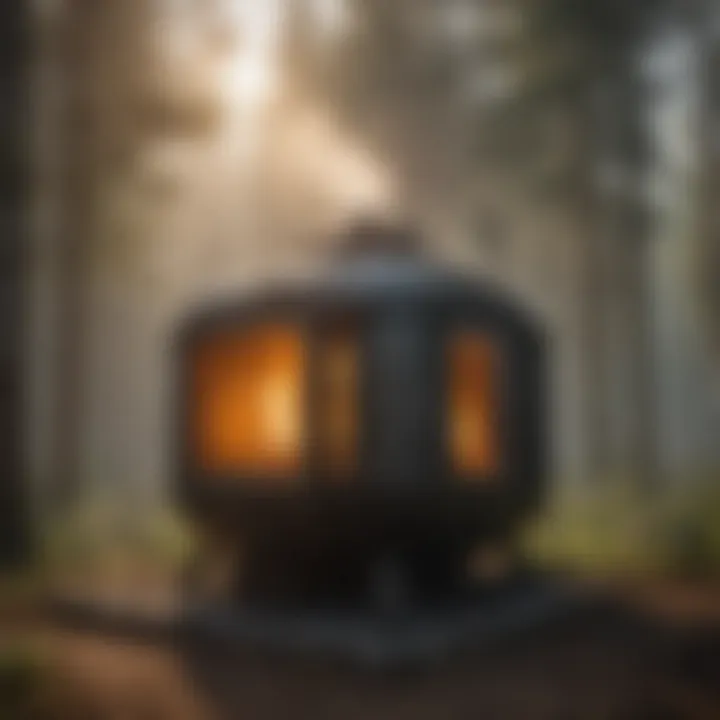
Intro
Biochar production is an increasingly prominent topic within the realm of sustainable forestry and soil management. The integration of biochar into forestry practices not only enhances soil health but also contributes to carbon sequestration strategies. Given the growing need for sustainable land management techniques, it is crucial to understand the equipment utilized in biochar production. This article will analyze the machinery involved, explore their functions, and assess their significance in achieving forestry objectives.
Understanding Forestry Practices
Forestry practices are essential in managing forests for ecological health, economic viability, and social benefits. It is important to recognize the different types of forestry methods that exist. These include:
- Clear-cutting: This involves removing all trees in a specific area, facilitating regeneration.
- Selective cutting: Only specific trees are harvested, allowing forest structure to remain intact.
- Shelterwood cutting: Trees are removed in phases, promoting natural regeneration under the protection of remaining trees.
The historical context of these practices dates back centuries, with various cultures implementing their own methods of forest management. Understanding these practices grounds the discussion in contemporary biochar production equipment. The advances in technology have shifted focus towards more sustainable practices, which includes the use of biochar.
Principles of Woodland Stewardship
Responsibility in managing woodland areas encompasses various conservation techniques. These techniques focus on maintaining biodiversity and ensuring that forest resources are used sustainably. Effective woodland stewardship principles include:
- Planting native species: This restores ecosystems and enhances biodiversity.
- Preventing soil erosion: Implementing effective soil conservation measures helps maintain soil structure and quality.
- Controlled burns: These clear underbrush and encourage new growth while reducing the risk of catastrophic wildfires.
Properly managing forest resources involves understanding the balance between harvesting and conservation. Equipment utilized for biochar production assists in this effort by transforming wood waste into a valuable resource, thereby reducing waste and promoting sustainability.
Sustainable Forest Management
Implementing sustainable forest management practices includes adhering to various certification standards. Organizations such as the Forest Stewardship Council (FSC) and the Sustainable Forestry Initiative (SFI) ensure practices meet environmentally sound criteria. These certifications attract eco-conscious consumers, creating market advantages for certified products.
The economic benefits of sustainability in forestry are significant. Investing in technology, like biochar production equipment, not only creates additional revenue streams but also enhances the overall health of the forest ecosystem. Producing biochar using waste materials reduces landfill costs and transforms waste into marketable products that enrich soil and sequester carbon.
"Sustainable forestry practices benefit both the environment and economy, creating a resilient future for forests and communities alike."
In summary, the production of biochar is inherently linked to improved forestry practices and sustainability. The equipment involved in its production plays a pivotal role in maintaining ecological balance, ensuring resource efficiency, and driving economic growth.
Prologue to Biochar Production
Biochar production represents a significant development in the realms of sustainable agriculture and environmental stewardship. This innovative process focuses on converting organic materials into a stable form of carbon that can enhance soil health and contribute to carbon sequestration. In the contemporary landscape, where climate change and soil degradation are pressing concerns, biochar offers a multifaceted solution, bridging the gap between waste management and sustainable usage of resources.
Understanding biochar production is not merely an academic exercise; it has real-world implications that affect farming practices, land management, and ecosystem restoration. Professionals in forestry and environmental sciences are increasingly recognizing the value of biochar equipment, as it facilitates the transformation of biomass waste into a resource that can rejuvenate soils and promote healthy plant growth. This section aims to lay the groundwork for comprehending biochar production's intricacies, its benefits, and the technologies involved.
Definition of Biochar
Biochar is a form of charcoal produced through pyrolysis, a process that involves heating organic material in the absence of oxygen. This carbon-rich product is characterized by its high porosity and surface area, which enhance its ability to retain water and nutrients in soil. By sequestering carbon, biochar serves as a tool for mitigating greenhouse gas emissions, making it a notable player in the fight against climate change. Its diverse applications range from soil amendment to waste management, underscoring its versatility.
Historical Context and Usage
The concept of biochar is not novel; it has roots in ancient civilizations. Historically, indigenous people in the Amazon Basin created terra preta, a nutrient-rich black soil that demonstrated the benefits of charcoal addition to agricultural lands. This ancient practice illustrates that the benefits of biochar for improving soil fertility and productivity have been recognized for centuries. In more recent years, biochar has gained attention due to its potential to improve modern agricultural systems, enhance carbon sequestration efforts, and contribute to sustainable land management practices.
As societies grapple with growing environmental concerns, revisiting historical practices such as biochar production is crucial. This perspective emphasizes the need for integrating traditional knowledge with contemporary technological advancements, paving the way for innovative solutions in biochar equipment and production methods.
The Science of Biochar
Understanding the science behind biochar is essential. It allows us to appreciate its role in environmental sustainability and agricultural efficiency. Biochar is not just a carbon-rich product; it encompasses a range of chemical and physical characteristics that contribute to its effectiveness. The detailed examination of these elements reveals how biochar can enhance soil quality, aid in carbon sequestration, and support sustainable forestry practices.
Chemical Composition
The chemical composition of biochar directly influences its behavior in soil and its utility in various agricultural applications. Biochar contains a high percentage of carbon, often exceeding 50% of its weight. This rich carbon content makes biochar a stable form of carbon, capable of persisting in soil for hundreds to thousands of years. In addition to carbon, biochar also includes essential nutrients such as nitrogen, phosphorus, and potassium, albeit in varying quantities depending on the feedstock used for its production.
The presence of minerals and trace elements is another important aspect of biochar's chemical composition. These can enhance the bioavailability of nutrients, thereby improving soil fertility. For example, potassium-rich biochars can boost crop yields in potassium-deficient soils. Moreover, the pH level of biochar can vary, impacting soil acidity and influencing plant growth.
Biochar's surface exhibits a porous structure with various functional groups. This porosity facilitates the absorption and retention of water and nutrients, an essential characteristic for promoting healthy plant growth. Additionally, the presence of carboxyl, hydroxyl, and carbonyl groups can interact with soil microorganisms, fostering beneficial soil ecology.
Physical Properties
Physical properties of biochar play a crucial role in determining its application effectiveness. One notable property is its bulk density, which is often lower than that of typical soil. This characteristic improves soil aeration and drainage, crucial for root growth. The porous structure of biochar also leads to high surface area, which contributes to the microbial habitat and enhances soil microbial activity.
Furthermore, biochar has a tendency to retain moisture, which is especially beneficial in arid regions. This moisture retention capability can reduce irrigation needs, leading to water conservation and more resilient agricultural systems. Various studies highlight that biochar amendments can significantly reduce soil erosion through improved structure.
In summary, the science of biochar is multifaceted, intertwining its chemical and physical properties. These elements contribute not only to soil and plant health but underscore the broader environmental benefits of biochar. By understanding these characteristics, forestry professionals and academics can make informed decisions about biochar application and its role in sustainable land management.
"Biochar is a powerful tool that bridges the gaps between carbon storage, soil enhancement, and sustainable agriculture. Its potential cannot be underestimated."
This shedding of light on biochar’s science lays the groundwork for better understanding of its production equipment and the broader implications for sustainable practices.
Types of Biochar Production Equipment
Understanding the types of biochar production equipment is essential for forestry professionals and academics. Each type has unique characteristics that affect the production process, efficiency, and the quality of the resulting biochar. This section offers detailed insights into various systems available, allowing readers to recognize the most suitable equipment for specific applications. The importance of selecting the right production equipment cannot be overstated; it determines not only the effectiveness of biochar production but also the economic feasibility and environmental impact associated with these operations.
Kiln-Based Systems
Kiln-based systems are one of the most traditional methods for producing biochar. These systems rely on direct heating with limited oxygen, facilitating the thermal decomposition of biomass materials. Common types of kilns include traditional earth kilns and modern industrial kilns. Each type has its advantages and disadvantages.
- Traditional Earth Kilns:
- Modern Industrial Kilns:
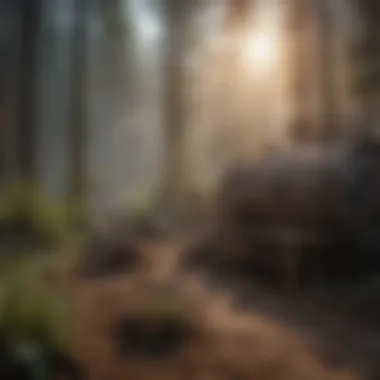
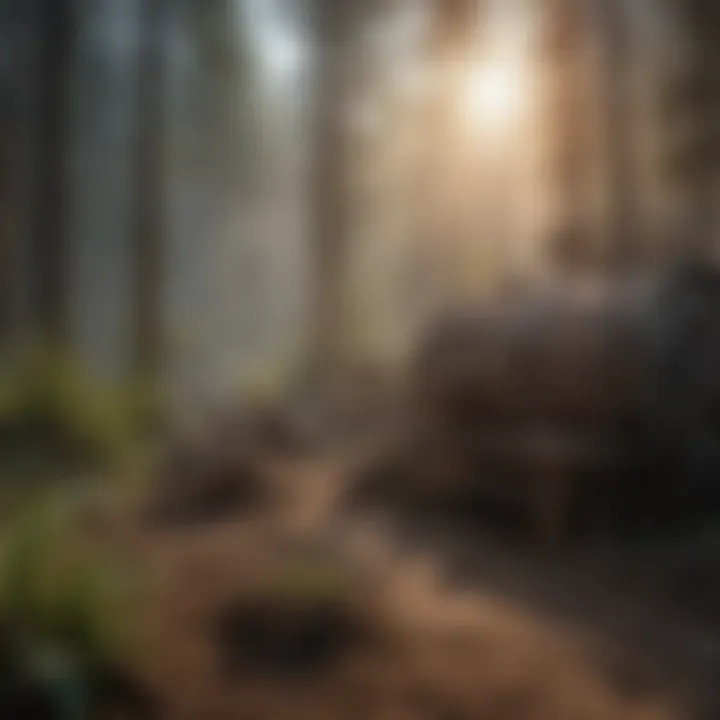
- Cost-effective and simple to construct.
- Suitable for small-scale production.
- Limited control over the carbonization process.
- Greater control over temperature and airflow.
- Enhanced efficiency, leading to higher yields of biochar.
- Suitable for larger operations.
Kiln-based systems may produce high-quality biochar, but they also need careful management to minimize emissions and ensure safety during operation. The simplicity of traditional kilns offers accessibility, while modern designs enable scalability and optimized outcomes.
Gasification Units
Gasification units represent an advanced method of biochar production, transforming solid biomass into gas through partial oxidation. This equipment allows for a more controlled environment and the possibility of energy recovery. Some key aspects include:
- Process Efficiency: Gasifiers can convert biomass into syngas, which can be used for electricity generation, thus making the process more economically viable.
- Dual Production: In addition to biochar, syngas can be used for heat or power generation.
- Environmental Impact: Gasification produces less particulate matter and lower greenhouse gas emissions compared to kilns.
These systems are often considered for industrial-scale operations due to their efficiency and potential energy recovery features. Still, the complexity and cost of gasification units may pose a challenge for small operators, necessitating careful economic consideration.
Pyrolysis Reactors
Pyrolysis reactors provide another method for biochar production, where biomass is thermally decomposed without oxygen, leading to the production of biochar, oil, and gas. These systems are characterized by:
- High Yields: Pyrolysis generally yields a higher percentage of biochar compared to other methods. This makes it attractive for those seeking quality.
- Temperature Control: Pyrolysis reactors allow for precise temperature adjustments, which can influence the properties of the resulting biochar significantly.
- Output Variation: Depending on the settings, pyrolysis can produce different ratios of biochar, bio-oil, and syngas, allowing for versatile applications in energy generation and soil amendment.
Although pyrolysis reactors can be capital intensive, their ability to optimize the products makes them a favored choice among larger operations aiming for quality and return on investment.
These production methods outline the landscape of biochar production equipment, each serving distinct needs and circumstances. Making informed decisions requires understanding these differences.
How Biochar Production Works
Understanding how biochar production works is crucial for maximizing its benefits within sustainable forestry and land management. This process involves two main stages: feedstock selection and preparation, followed by the carbonization process. Each stage carries significance in determining the efficiency of biochar production and the quality of the final product.
Feedstock Selection and Preparation
Feedstock is the raw material used to produce biochar. Choosing the right feedstock is essential. Various organic materials can be utilized, including wood chips, agricultural residues, and even animal manures. However, the chemical and physical properties of the chosen feedstock significantly impact the properties of the resulting biochar.
A well-prepared feedstock promotes better conversion efficiency and produces biochar with desirable characteristics. Here are some key considerations:
- Moisture Content: The moisture level in the feedstock affects the energy required for carbonization. Ideally, the moisture content should be below 20%, ensuring optimized thermal conversion.
- Particle Size: Smaller particles generally enhance heat transfer during the carbonization process. Materials should be chipped or ground to achieve uniform sizes for best results.
- Contaminants: Avoiding feedstock with contaminants, like plastics or chemicals, is important for producing quality biochar. Cleanup processes may be necessary to ensure purity.
By addressing these factors, producers can ensure that the feedstock yields high-quality biochar, enhancing its effectiveness in soil health improvement and carbon sequestration.
Carbonization Process
The carbonization process involves heating the prepared feedstock in an oxygen-limited environment. This thermal decomposition results in the conversion of the biomass to stable carbon, known as biochar. There are multiple methods for carbonization, and each brings its own specific benefits. Here are critical aspects:
- Temperature: The temperature at which carbonization occurs determines the characteristics of biochar. Higher temperatures promote greater carbon stability but reduce the yield of biochar. Optimal temperatures typically range from 300°C to 700°C.
- Duration: The time spent in the carbonization phase can also influence biochar's properties. Shorter durations result in different chemical profiles compared to longer carbonization times, affecting its efficacy as a soil amendment.
- Type of Equipment: The choice of carbonization equipment—be it kilns, gasifiers, or pyrolysis reactors—affects both efficiency and product quality. Each technology has its own operational parameters that dictate performance and output.
"The carbonization process is where biomass transforms into biochar, which carries essential properties beneficial for soil health."
Operational Considerations
Operational considerations are critical in the context of biochar production equipment. They encompass various aspects that ensure efficient, safe, and sustainable production processes. Understanding these considerations can greatly enhance the performance of biochar production systems, ultimately benefiting both operators and the environment.
Energy Requirements
The energy requirements of biochar production equipment can vary significantly depending on the technology used. For instance, pyrolysis reactors may require substantial energy to reach the required temperatures for efficient carbonization. Efficient energy management is essential to minimize operating costs.
It is important to assess the type of energy sources available. Many facilities use renewable energy, such as solar or bioenergy, to power their operations. Using sustainable energy reduces the carbon footprint of biochar production. Additionally, capturing waste heat from the process can improve energy efficiency.
Safety Measures
Safety measures are paramount in any biochar production setup. Due to high temperatures and potentially hazardous materials involved, implementing protocols to protect workers and the environment is essential. Proper training for personnel on equipment operation and emergency protocols can reduce accidents dramatically.
Some safety measures include:
- Proper Ventilation: Ensure that all equipment has adequate ventilation to prevent harmful gas accumulation.
- Fire Safety: Install fire extinguishers and other firefighting equipment nearby. Conduct regular fire drills to prepare staff.
- Protective Gear: Provide operators with necessary protective clothing and equipment to minimize exposure to heat and chemicals.
Effective safety measures not only protect personnel but also enhance operational uptime and efficiency.
Maintenance Protocols
Regular maintenance protocols are vital to ensure the longevity and efficiency of biochar production equipment. Unattended machinery can lead to breakdowns, causing production delays and increased costs.
Key maintenance tasks include:
- Routine Inspections: Schedule regular checks of equipment components to identify wear and potential issues.
- Cleaning Systems: Build up of ash or biochar residues can affect equipment performance. Implement cleaning schedules to maintain optimal performance.
- Documentation: Keep records of all maintenance activities. This data can help predict and prevent future issues.
Incorporating these operational considerations into biochar production will not only improve the effectiveness of the equipment but also contribute to safer, more sustainable practices.
Economic Implications of Biochar Production
Understanding the economic implications of biochar production is crucial in assessing its viability as a solution for sustainable forestry and soil management. This section examines the financial aspects that are intertwined with the production of biochar, highlighting the costs associated with equipment, the market demand, and the potential for revenue generation. As biochar garners attention for its environmental benefits, the economic angle becomes increasingly relevant in the decision-making processes of both producers and policymakers. Evaluating these aspects helps stakeholders understand the financial landscape and encourages investment in biochar technology.
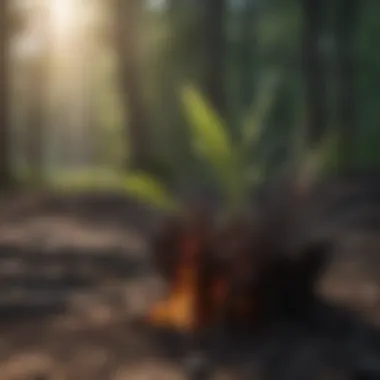
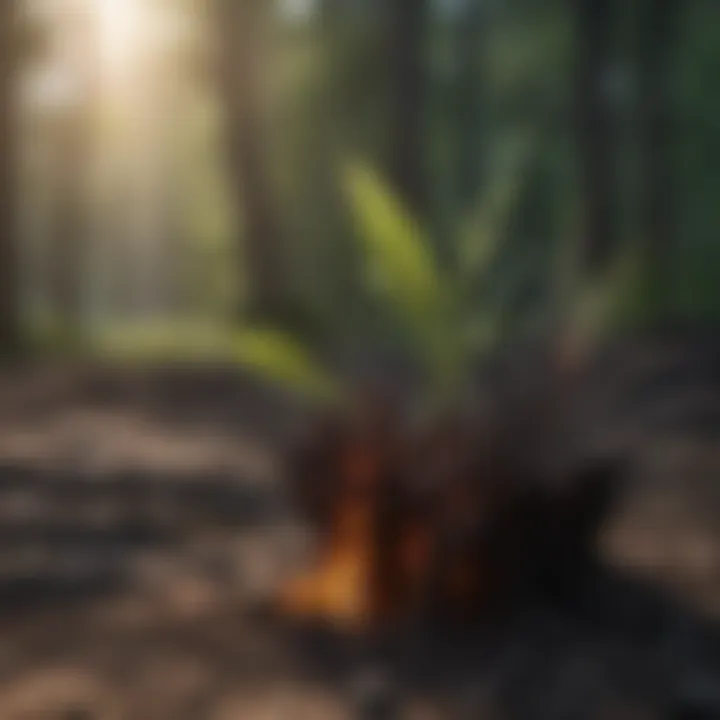
Cost Analysis of Equipment
Cost analysis is a vital component when deciding to engage in biochar production. The type of equipment selected plays a significant role in the overall financial outcome. Different production equipment have their own price tags, maintenance requirements, and operational costs. For instance, pyrolysis reactors often require a higher initial investment but provide a more efficient carbonization process, potentially leading to greater yields of biochar compared to simpler kiln-based systems.
Among these considerations, operational expenses such as energy consumption, feedstock preparation, and labor costs must also be evaluated. Moreover, considering the warranty and lifespan of the equipment provides insights into long-term financial commitments.
- Initial Costs:
- Operating Costs:
- Financing Options:
- Equipment purchase price
- Installation expenses
- Maintenance fees
- Energy costs
- Leasing versus buying
- Grants and subsidies available
The total cost analysis permits stakeholders to create a comprehensive budget, ultimately guiding them in making informed choices regarding their engagement in biochar production.
Market Demand and Revenue Potential
The market demand for biochar has seen a notable increase, driven by its applications in agriculture, landscaping, and environmental management. The growing recognition of biochar's capabilities in enhancing soil quality, retaining moisture, and sequestering carbon are contributing factors to this rising demand. Studies suggest that adopting biochar can lead to improvements in crop yields, which intrigues farmers and land managers looking to optimize their production methods.
The revenue potential associated with biochar production also requires close inspection. Selling biochar as a soil amendment can establish a profitable revenue stream. Additionally, partnerships with local governments or non-profits promoting sustainable practices can open avenues for subsidies, contracts, or co-financing arrangements.
- Potential Applications of Biochar:
- Soil amendment
- Wastewater treatment
- Carbon credits trading
Biochar's integration into existing markets, along with future trends in environmental conservation, suggest a promising outlook for producers who embrace these opportunities. The economic implications outlined in this article underscore the importance of not only understanding the costs associated with equipment but also the opportunities present in a rapidly evolving market.
Environmental Benefits of Biochar
Biochar stands as a pivotal element in the realm of environmental sustainability. This section investigates how biochar contributes positively to ecosystems, particularly focusing on soil health and carbon sequestration. With growing concerns about climate change and soil degradation, the role of biochar is increasingly regarded as beneficial.
Soil Health Improvement
Biochar is known for enhancing soil health through its unique properties. It improves soil structure, which promotes better aeration and water retention. The porous nature of biochar creates habitat for beneficial microorganisms, thereby fostering a thriving soil ecosystem.
- Nutrient Retention: Biochar has a high cation exchange capacity, meaning it can hold nutrients within the soil, making them available for plant uptake.
- pH Regulation: It can help in adjusting the pH of acidic soils. This is crucial for many crops and plants that require a specific pH range for optimal growth.
- Reduced Soil Erosion: The addition of biochar helps bind soil particles together, reducing erosion and loss of topsoil, which is vital for sustainable agriculture.
In summary, biochar does not merely act as a supplement. It rejuvenates the very foundation of soil, leading to healthier ecosystems and better crop yields.
Carbon Sequestration Potential
Another significant aspect of biochar lies in its ability to sequester carbon. The carbon that constitutes biochar remains stable for hundreds to thousands of years in the soil, effectively removing carbon dioxide from the atmosphere.
- Long-Term Storage: There is evidence that biochar can stabilize carbon. When applied to soil, approximate 50-60% of its carbon content can persist for very long periods, thus contributing to a reduction in greenhouse gases.
- Complement to Other Practices: Integrating biochar with practices like agroforestry can amplify its impact on carbon sequestration. When combined, these methods can lead to enhanced carbon storage within both soil and biomass.
- Mitigating Climate Change: By producing and utilizing biochar, we can potentially offset a portion of the greenhouse gases emitted annually, thereby offering a tangible action towards climate change mitigation.
"The production and application of biochar provide unique solutions to some of the most pressing environmental challenges."
Technological Advancements in Biochar Equipment
Technological advancements have significantly impacted the production of biochar in various ways. Increased efficiency, reduced environmental footprint, and enhanced product quality are some of the noticeable benefits of these innovations. As biochar garners attention for its potential in sustainable forestry and soil management, understanding these advancements is crucial for stakeholders in the industry.
Innovative Manufacturing Techniques
Innovative manufacturing techniques play a vital role in the development of biochar production equipment. One notable technique is the development of continuous feed systems. These systems enable the uninterrupted input of feedstock, which results in more efficient processing. They reduce downtime and increase overall output.
Other innovations include modular designs that allow for scalability. Such designs enable operations to adjust their production capacity based on demand. This flexibility is particularly useful for small to medium enterprises, allowing them to expand gradually without prohibitive investment.
Additionally, advanced thermal processes, including torrefaction and hydrothermal carbonization, have emerged. These techniques create biochar under specific conditions that optimize yield and enhance biochar characteristics. For instance, compared to traditional methods, hydrothermal carbonization can lead to higher carbon content and better nutrient retention in the biochar produced, making it more effective as a soil amendment.
Automation and Efficiency
Automation has revolutionized the biochar production landscape. The integration of digital technologies allows for real-time monitoring and control of processes, ensuring consistent quality in production. Automated systems can adjust parameters like temperature and pressure dynamically, leading to optimized conditions for carbonization and minimizing human error.
Moreover, innovative sensor technologies help in gauging feedstock quality and moisture levels. This data can guide adjustments to the process, ensuring the best possible output.
"Automation in biochar production not only enhances efficiency but also contributes to resource conservation."
Finally, energy recovery systems are now more prevalent in biochar production. These systems capture excess heat generated during the carbonization process, which can be reused in the system or converted to electricity. This not only reduces operational costs but also lowers the carbon footprint associated with biochar production. Efficiency in production thus not only pertains to volume but also to resource utilization, which is essential as the world searches for sustainable practices.
In summary, technological advancements in biochar equipment have made production more efficient and sustainable. By exploring innovative manufacturing techniques and the impact of automation, industry professionals can better understand and take advantage of these tools to meet the increasing demand for biochar.
Case Studies in Biochar Production
Case studies provide valuable insights into real-world applications of biochar production equipment. By examining successful projects, we can uncover best practices, understand challenges, and highlight the significant advantages of biochar in various contexts. These examples allow us to see how theoretical knowledge translates into practical implementations, which is essential for forestry professionals seeking to adopt similar strategies in their own work.
Successful Implementation in Forestry
One compelling case study is the implementation of biochar technology in forestry management. In regions where deforestation poses a significant threat, biochar production can contribute to sustainable practices. For instance, a forestry company in the Pacific Northwest utilized waste biomass from logging activities to produce biochar. By reducing slash piles that traditionally contribute to emissions when burned, they not only created a valuable soil amendment but also improved soil health on their forest lands.
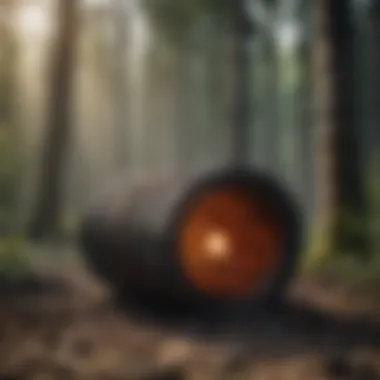
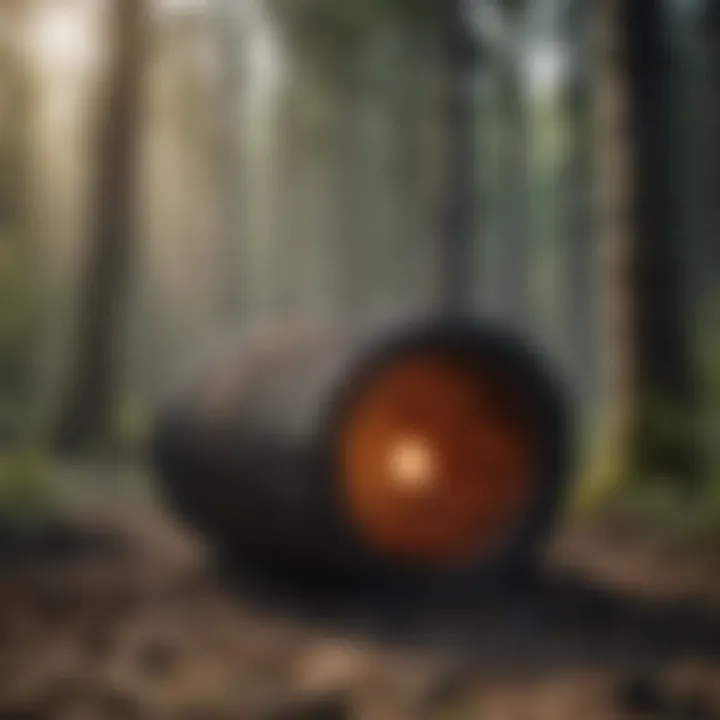
Benefits noted from this implementation include:
- Enhanced soil fertility, leading to better tree growth.
- Reduction in greenhouse gas emissions from waste disposal.
- Increased carbon sequestration through the application of biochar in soil.
The project demonstrated that integrating biochar production into forestry operations can create a more sustainable cycle of resource use. The results were measurable, showing improved growth rates of planted tree species and a significant decrease in the company's overall carbon footprint.
Community-Based Projects
Community-based projects are also noteworthy in the context of biochar production. An example can be found in a small town in New England where local organizations collaborated to promote soil health and sustainability. Residents utilized yard waste and agricultural residues to create biochar, which they applied to community gardens and small-scale farms.
This initiative had multiple benefits, including:
- Improved soil structure and moisture retention in residential gardens.
- Educated community members on sustainable practices and waste utilization.
- Fostered a sense of community involvement centered on environmental stewardship.
Moreover, this case highlighted the scalability of biochar projects. Small-scale efforts can yield significant improvements in local environments while engaging community members in sustainable practices.
"Localized projects demonstrate that even small contributions to sustainable practices can lead to larger environmental benefits."
As we analyze these case studies, it becomes clear that biochar production is not merely a technological advancement but rather a multifaceted approach to address ecological challenges. Incorporating biochar practices into forestry operations and community projects exemplifies how collective efforts can foster innovation and sustainability in environmental management.
Challenges in Biochar Production
The production of biochar, while beneficial for many environmental and agricultural practices, faces a range of challenges that can impede its progress and acceptance. These challenges can be broadly categorized into regulatory hurdles and the public perception surrounding biochar. Addressing these issues is crucial for the broader adoption of biochar production equipment and practices. As such, understanding these challenges provides valuable insight for forestry professionals, policymakers, and environmental advocates.
Regulatory Hurdles
Regulatory hurdles play a pivotal role in the development and implementation of biochar production technologies. These can include zoning laws, environmental regulations, and local policies governing waste management. The variation in regulations can lead to confusion and may deter investment in biochar projects.
Each geographical region has its own set of rules that dictate what is permissible in terms of biomass feedstock, production methods, and the resulting biochar's applications. Often, these regulations are not entirely clear or lack comprehensiveness, which can lead to uncertainty among producers about compliance. Moreover, obtaining necessary permits can be a time-consuming affair, potentially delaying projects and increasing costs. For instance, regulatory approvals may take several months or even years, particularly if there is significant pushback from local communities or environmental activists.
Another regulatory challenge arises from the lack of standardized testing methodologies to evaluate biochar products. Without clear guidelines for quality assessment, it can be challenging to establish trust and reliability in biochar's ecological claims. As a result, various entities may set different thresholds for what constitutes acceptable biochar, complicating market entry for new producers.
Public Perception and Acceptance
Public perception significantly influences the adoption of biochar production. Many communities may hold misconceptions about the processes involved, particularly concerning emissions and environmental impacts. People often worry about the potential release of harmful substances during production, despite scientific evidence supporting safe production practices.
The skepticism surrounding biochar stems from anecdotal experiences and historical precedents. Communities that have encountered negative outcomes related to similar technologies may generalize these fears to biochar, which is unfair and unfounded. It is important to educate the public on the benefits of biochar, such as improved soil health and carbon sequestration potential.
Community engagement can, therefore, serve as a tool for raising awareness about biochar. Collaborating with local stakeholders, providing transparent information, and showcasing successful case studies can mitigate fears and encourage acceptance. Furthermore, fostering an open dialogue between producers and local communities can help build trust and support for biochar initiatives.
Getting beyond these challenges requires tenacious effort from all involved parties. By navigating regulatory frameworks and addressing public concerns, the biochar industry can move forward, realizing its significant potential while promoting sustainable practices in forestry and agriculture.
"Overcoming challenges in biochar production is essential to leveraging its full benefits for sustainable practices across various sectors."
Future Trends in Biochar Technology
The future of biochar technology holds significant promise as it continues to evolve. This section addresses the importance of integrating innovative approaches and new research initiatives to enhance the efficiency and effectiveness of biochar production. As stakeholders in forestry and sustainability look for better solutions, understanding these trends becomes essential for guiding future practices and policies.
Research Directions
Current research in biochar technology focuses on several key areas. One major direction is the optimization of feedstock. Different types of biomass can yield varying quality of biochar, thus researchers are studying optimal combinations and treatment processes. Another area of investigation is the modification of production processes, such as improving pyrolysis techniques or developing faster and more energy-efficient methods.
In addition, there is increasing interest in the mathematical modeling of biochar production. This can help predict outcomes based on different variables, aiding in scalability and effective resource management.
While examining the impact on soil, new studies are exploring how varying particle sizes or application methods affect plant growth and nutrient retention. Overall, ongoing investigations will pave the way for a more systematic understanding of biochar's potential uses and impacts.
Integration with Other Sustainable Practices
Integrating biochar production with other sustainable practices is essential for maximizing its benefits. For example, combining biochar application with sustainable agriculture can enhance soil fertility while reducing dependence on chemical fertilizers. Additionally, partnerships between biochar producers and local agriculture can stimulate community economies while promoting environmental sustainability.
Moreover, biochar's role in carbon credits and climate action plans must not be overlooked. Aligning biochar strategies with climate goals provides incentives for more widespread adoption.
Therefore, aligning biochar technology with practices such as composting, agroforestry, and permaculture not only enhances its effectiveness but also contributes to holistic sustainable development. By paying attention to these integrations, forestry professionals can leverage biochar production as a component of broader ecological objectives.
"As biochar technology evolves, the ability to combine multiple sustainability strategies could create synergies that amplify environmental benefits."
Finale
Ending
The conclusion of this article brings together the key findings and insights regarding biochar production equipment. It emphasizes the multifaceted role that biochar plays in sustainable forestry and soil management. Understanding this topic is crucial for forestry professionals and academics as it encapsulates the significance of equipment in the biochar production process, which leads to a variety of ecological and economic benefits.
Summary of Key Points
In summarizing the key points, we can highlight the following:
- Biochar is a carbon-rich form of charcoal produced through the pyrolysis of organic materials. It enhances soil health, promotes carbon sequestration, and improves agricultural productivity.
- Various types of biochar production equipment, including kiln-based systems, gasification units, and pyrolysis reactors, each have unique operational methodologies and advantages.
- The production process involves careful feedstock selection, carbonization, and operational considerations, including energy efficiency and maintenance regimes.
- Economic implications underscore both the costs associated with biochar production equipment and the revenue potential through market demand for biochar as a soil amendment.
- Environmental benefits, particularly regarding soil health improvement and the role of biochar in mitigating climate change, are significant in the discussion of sustainability.
- Technological advancements in biochar production equipment are continually evolving, with innovative techniques enhancing automation and efficiency.
- Challenges, including regulatory hurdles and public perception, require attention to fully realize the potential of biochar production.
Final Thoughts on Biochar Production Equipment
In concluding, the narrative surrounding biochar production equipment is a complex one that intertwines technology, environmental science, and economic feasibility. The advancements in production methods not only cater to the increasing demand for sustainable practices but also raise awareness of the environmental challenges we face today.
Addressing the complexities involved in biochar production equipment is essential. It offers opportunities to increase soil health, contribute to carbon sequestration efforts, and enhance the viability of forestry practices. The intersection of these elements signifies a profound responsibility for forestry professionals and stakeholders in land management.
"Biochar has the potential to bridge the gap between sustainability and productivity in forestry practices. Its strategic use can lead to long-term environmental benefits, making it a valuable ally in our ecological endeavors."
In light of these considerations, the future of biochar production equipment looks promising. Continuous research and adaptation to emerging technologies will play a pivotal role in its evolution, shaping the future of sustainable land management.